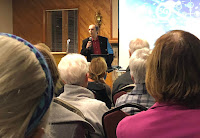 |
5G Town Hall
Rochester, Michigan
November 7, 2019. |
Yesterday I participated in a town hall meeting in
Rochester, Michigan to discuss the new 5G cell phone technology. I was invited to attend in part because of my contributions to the book
Intermediate Physics for Medicine and Biology, which discusses the health risks of electromagnetic fields.
When preparing for the event, I created a list of frequently asked questions (well, these were the questions I thought people would ask). Not wanting to waste this effort, I reproduce my
FAQ below.
The event was....interesting. I was impressed by the passion of these concerned citizens, who packed a large room on a cold Thursday evening and for over two hours asked questions and voiced their opinions (mostly voiced their opinions). I’ve taught plenty of apathetic 20-something-year-olds who don’t engage with the lecture or challenge what I say, so I found this feisty crowd refreshing. Unfortunately, I was not convinced by their claims of dire health effects from 5G technology, and they were not convinced by me. The most disturbing moment was when I said something along the lines of “if you want to know more about the risks of cancer, consult the
National Cancer Institute” and the response was a chorus of “No, No, No!” Goodness, if we can’t trust the National Cancer Institute to understand cancer, who can we trust? But no one threw a tomato at me, so I’ll call the evening a success. The FAQ below summarizes my view on this matter.
FAQ: 5G Cell Phone Health Effects
What is 5G?
5G is the fifth generation of technology for
cell phones. It uses higher frequencies of
electromagnetic radiation than 4G technology (up to 300
GHz, with a wave length of 1
mm).
What does IPMB say about the health risks from cell phones?
Section 9.10.5 of
Intermediate Physics for Medicine and Biology addresses possible health risks from microwaves, mobile phones, and wi-fi.
Russ Hobbie and I cite a
2005 review by
John Moulder,
Ken Foster, and their colleagues, which concludes that “Overall, a weight-of-evidence evaluation shows that the current evidence for a causal association between cancer and exposure to
RF [radiofrequency] energy is weak and unconvincing.” We also cite “an exhaustive (390 page) report…by the
International Committee on Non-Ionizing Radiation Protection (
Vecchia et al. 2009)” that reaches a similar conclusion. Next we write that “
Sheppard et al. (2008) evaluated all the proposed mechanisms for radio-frequency interactions with biological molecules and processes…[and conclude that] the principal mechanism for biological effects, and the only well-established mechanism, is the heating of tissues.” Finally, “
Foster and Moulder (2013) reviewed the current state of research [on the health effects of wi-fi, and conclude that the evidence provides] ‘no basis to anticipate any biological effects.’”
The 2013 review by Foster and Moulder summarizes my opinion on this topic.
“Impossibility”
arguments are difficult to sustain in biology; but the lack
of a generally-accepted mechanism by which low-level
(below ICNIRP and IEEE limits) RF fields in the GHz frequency
range could produce biological effects, after many
years of sustained efforts to uncover such mechanisms,
makes it increasingly unlikely that any mechanism will be
found.
What have Foster and Moulder said lately about health risks from wi-fi?
Ken Foster and John Moulder are experts on the interaction of electromagnetic radiation with the body. They are skeptical about many claims of health risks caused by power-line, cell-phone, and wi-fi radiation. Both are now emeritus professors; Foster at the
University of Pennsylvania and Moulder at the
University of Wisconsin.
Intermediate Physics for Medicine and Biology cites Foster and Moulder several times, but the 5th edition of
IPMB was published in 2015. What have they said lately?
Foster published a
post two months ago in a
Scientific American blog looking specifically at 5G technology and health risks. His conclusion: “So far, at least, there’s little evidence of danger.”
The most interesting development is a
critique of Foster and Moulder’s 2013
review by
Martin Pall, an emeritus professor at
Washington State University. He concludes that “there are seven repeatedly found Wi-Fi
effects which have also been shown to be caused by other similar EMF [electromagnetic field] exposures. Each of the seven should be
considered, therefore, as established effects of Wi-Fi.”
Pall’s central hypothesis is that cell phone radiation affects calcium ion channels, which if true could trigger a cascade of biological effects. In a
rebuttal, Foster and Moulder (2018) write
Pall (2018) criticizes our 5-year-old review of studies related to Wi-Fi and health (Foster and Moulder 2013). We respond to his critique, and also note weaknesses in his selection and interpretation of studies on biological and health effects of Wi-Fi type signals...
Having examined the additional papers that Pall cites, we reaffirm our earlier conclusion: a number of studies have reported bioeffects of Wi-Fi exposures, but technical limitations make many of them difficult to interpret and artifacts cannot be excluded. We are not aware of any health-agency warnings about health risks of Wi-Fi technology. Despite some level of public controversy and an ongoing stream of reports of highly variable quality of biological effects of RF energy (e.g. articles in a recent special issue of the Journal of Chemical Neuroanatomy, Volume 75, 2016) health agencies consistently conclude that there are no proven hazards from exposure to RF fields within current exposure limits (even as they consistently call for more research).
My advice is to read the review, the critique, and the rebuttal, and then draw your own conclusion. You may find many of the technical details difficult to understand (I do), but you will better appreciate the complexity if these issues, and the difficulty in drawing definite conclusions from imperfect data. I don’t agree with Pall’s claims.
What does Bob Park have to say about the health risks from cell phones?
Robert Park is an emeritus professor of physics at the
University of Maryland, and was the director of public information at the Washington office of the
American Physical Society. He is the author of
Voodoo Science, and wrote a weekly column titled “What’s New” debunking
pseudoscience. His health has not allowed him to contribute to the recent discussion about the risks of cell phones and 5G technology (and, oh, how we miss him). Here’s what he wrote in “What’s New” on Sunday, May 6, 2012.
1. ALBERT WHO? DEAD PHYSICIST DISPELS MOBILE-PHONE MYTH.
According to news reports last week: "There is still no evidence of harm to health from mobile-phone technologies," or other wireless devices such as Wi-Fi. A study for the UK's Health Protection Agency (HPA) is said to be the most complete review yet and new evidence is still being examined, according to Professor Anthony Swerdlow of the Institute of Cancer Research, who chaired the study. I once had a rubber stamp made that said: “More research is needed,” since its found at the end of every science paper. The unanswered question is why anyone thought microwave radiation might be a cancer agent in the first place? Cancer is linked to the formation of mutant strands of DNA. More than 100 years ago in his 1905 paper on the photoelectric effect, Albert Einstein predicted an abrupt threshold for photoemission at about 5 eV, just above the lovely blue limit of the visible spectrum, demonstrating wave-particle duality. He was awarded the 1923 Physics Nobel Prize [actually, Einstein received the Nobel Prize in 1921, and Robert Millikan received it in 1923, in part for his experimental work on the photoelectric effect]. Its also the threshold for the emission of invisible ultraviolet radiation that causes hideous skin cancers. The cancer threshold, is therefore, 1 million times higher than the microwaves band. The same enormous mistake was made in the 1980s when epidemiologists falsely warned that exposure to power line emission can cause cancer. Power lines abruptly stopped causing cancer in 1997 after the U.S. National Cancer Institute conducted a better study. Its painful to witness this sad history being replayed with mobile-phone radiation. Aside: My apologies to regular readers who have heard this 20 times before, but it has not gotten through to everyone.
Park’s argument remains as true now as eight years ago, except that a 300 GHz photon has an energy of one-thousandth of an electron volt, which is “only” a few thousand times less than the threshold for photoemission or UV skin cancer (and is about 25 times smaller than thermal energy, 0.025 eV). The possibility of DNA damage—the underlying cause of cancer—remains extraordinarily remote, but not as ridiculously remote as it was for cell phone technology ten years ago.
Electromagnetic radiation is considered a possible carcinogen. What’s that mean?
Something that is “possibly carcinogenic to humans” doesn’t probably cause cancer. It probably doesn’t cause cancer, but we can’t say for certain. RF radiation was
not placed into two more threatening categories: “probably carcinogenic to humans” and “carcinogenic to humans.”
The website
Science-Based Medicine states that
Despite the negative evidence to date, in 2011, the International Agency for Research on Cancer classified EMF [low-frequency electromagnetic fields] as a “possible” carcinogen. They have a low threshold for this category, which is rather long. It requires limited evidence of carcinogenic potential in humans and inadequate evidence in animals. This is the, “Probably should do more research just to be sure, but basically don’t worry about it,” category.
Who was Eleanor Adair, and what did she think about microwaves?
I have
written about Eleanor Adair before in this blog. She was a leading expert on the interaction of microwaves with biological tissue, and was skeptical of any health hazards claims. A
New York Times interview included this exchange:
Q. If I were to say to people, “Hey there’s this really cool idea: Why heat your whole house when you could use microwaves to heat yourself?” they would say: “You’ve got to be kidding. Don’t you know that microwaves are dangerous? They can even cause cancer.” What do you say to people who respond like that?
A. I try to educate them in exactly what these fields are. That they are part of the full electromagnetic spectrum that goes all the way from the radio frequency and microwave bands, through infrared, ultraviolet, the gamma rays and all that.
And the difference between the ionizing X-ray, gamma ray region and the microwave frequency is in the quantum energy. The lower you get in frequency the lower you get in quantum energy and the less it can do to the cells in your body.
If you have a really high quantum energy such as your X-rays and ionizing-radiation region of the spectrum, this energy is high enough that it can bump electrons out of the orbit in your cells and it can create serious changes in the cells of your body such that they can turn into cancers and various other things that are not good for you.
But down where we are working, in the microwave band, you are millions of times lower in frequency and there the quantum energy is so low that they can’t do any damage to the cells whatsoever. And most people don’t realize this.
Somehow, something is missing in their basic science education, which is something I keep trying to push. Learn the spectrum. Learn that you’re in far worse shape if you lie out on the beach in the middle of summer and you soak up that ultraviolet radiation than you are if you use your cell phone.
Any new data about health effects of electromagnetic fields in the last few years?
A recent article examining the “
Occupational Exposure to High-Frequency Electromagnetic Fields and Brain Tumor Risk in the INTEROCC Study: An Individualized Assessment Approach,” (Vila et al., 2018) provides the following highlights
• Evidence on health effects of long-term occupational exposure to high-frequency EMF remains weak
• Individualized cumulative occupational RF [radiofrequency, 10 MHz–300 GHz] and IF [intermediate frequency, 3 kHz–10 MHz] exposure indices were assigned to study subjects
• No clear associations with RF or IF EMF and glioma or meningioma risk were observed
• The possible role of RF magnetic fields on brain tumor promotion/progression should be further investigated.
As Bob Park said, everyone supports doing additional research (as do I). But I don’t see a lot to be worried about here.
Bill Curry concluded that 5G technology “is likely to be a serious health hazard.” Well?
I’ve written about physicist Bill Curry and his claims
previously in this blog. That post begins
A recent article by William Broad in the New York Times—titled “The 5G Health Hazard That Isn’t”—tells the sad story of how unfounded fears of radiofrequency radiation were stoked by one mistaken scientist.
What is electromagnetic hypersensitivity?
Some people claim they’re extremely sensitive to weak electromagnetic fields. The SkepDoc
Harriet Hall wrote a blog post titled “
Myths About Electromagnetic Hypersensitivity and Multiple Chemical Sensitivity.” She begins
As if we didn’t have enough things to worry about already, now we are being told to fear our toasters. A typical headline trumpets “The Effects of Invisible Waves.” We are increasingly exposed to electromagnetic radiation from cell phones, cell phone towers, wireless Internet routers, cordless phones, and power lines. Other sources ... are our household appliances: TVs, hairdryers, light bulbs, and yes, your trusty toaster. These invisible villains are said to lead to a variety of symptoms, including poor sleep, fatigue, heart palpitations, headache, nausea, dizziness, memory impairment, prickling and burning sensations, along with skin rashes. They’ve even been blamed for depression, anxiety, colds, digestive disorders, and chronic pain. It’s called electromagnetic hypersensitivity or EHS.
Hall concludes
The symptoms described by “electromagnetic hypersensitivity” sufferers can be severe and are sometimes disabling. However, it has proved difficult to show under blind conditions that exposure to EMF can trigger these symptoms. This suggests that “electromagnetic hypersensitivity” is unrelated to the presence of EMF.
I recommend you read the entire article.
IPMB cited a point-counterpoint article that suggests cell phones are dangerous. True?
Point-counterpoint articles appear every month in the journal
Medical Physics. They are a wonderful teaching tool, allowing students to consider and discuss questions at the cutting edge of medical physics. The one cited in Chapter 9 of
IPMB is by Khurana, Moulder, and Orton (2008), titled “
There is Currently Enough Evidence and Technology Available to Warrant Taking Immediate Steps to Reduce Exposure of Consumers to Cell-Phone-Related Electromagnetic Radiation.” Every point-counterpoint article pits one researcher against another, arguing opposing sides of the claim made in the article title. In this case,
Vini Khurana supports the proposition, and John Moulder opposes it;
Colin Orton is the moderator. I encourage you to read the article for yourself. I agree with Moulder’s conclusion that
weak epidemiological evidence of an association of mobile phone use with brain cancer incidence, when combined with the biophysical implausibility of a causal link and the strongly unsupportive animal studies, does not support the case that regulation of mobile phone use is urgently needed.
Last year I saw an article that says 5G cell phone radiation is unsafe! What’s up?
The Nation published an article titled “
How Big Wireless Made Us Think That Cell Phones Are Safe: A Special Investigation. The Disinformation Campaign—and Massive Radiation Increase—Behind the 5G Rollout,” by
Mark Hertsgaard and
Mark Dowie.
David Gorski published a
critique of this article for the website
Science-Based Medicine. He writes
The Nation indulges in fear mongering about cell phones and cancer
An article published last week in The Nation likens wireless telephone companies to tobacco and fossil fuel episodes in their tactics of spreading fear, misinformation, and doubt regarding the science of cell phone radiation and health. To produce this narrative, the investigation’s authors rely on unreliable sources and cherry pick scientific studies, ignoring the scientific consensus that cell phone radiation almost certainly doesn’t cause cancer, all the while disingenuously claiming that they aren’t taking a position on the health effects of radio waves.
Read
The Nation article and the critique and decide for yourself. At the least, you’ll learn how physics can be applied to medicine and biology.
What’s the bottom line regarding the risk of cancer from 5G cell phones?
Electromagnetic radiation consists of packets of energy called
photons. The energy of a photon increases with the frequency of the radiation.
Cancer is caused when DNA is damaged by very-high-frequency photons, such as
x-rays (
ionizing radiation). If the frequency is in the range of 300 GHz, the energy of a photon is far too small to disrupt bonds in DNA. It is, in fact, smaller than the energies associated with thermal motion of molecules. So, photons associated with 300 GHz radiation cannot cause cancer by damaging DNA. Of course, you could have a whole lot of 300 GHz photons, and they might pool their effort and together have enough energy to break bonds. We have a word for that:
heat. 300 GHz radiation can heat tissue, but such heating is well understood and easily measured; Cell phone radiation is too weak to cause a significant temperature increase. So, we are left with no plausible mechanism for health risks from cell phone radiation. Perhaps some secondary effect could make your body less able to fight off cancer once it is induced by other mechanisms, but no one really knows how that might occur. Moreover, the
epidemiological evidence suggests there is little risk. Cell phone use has increased dramatically since the turn of the century, but the incidence of brain cancer hasn’t increased. The
National Cancer Institute says “
The only consistently recognized biological effect of radiofrequency radiation in humans is heating... There are no other clearly established effects on the human body from radiofrequency radiation.” I wouldn’t say it’s impossible that cell phones put you at risk for cancer, but it’s unlikely. In my opinion, it’s exceedingly unlikely. We have many other things to worry about instead.
References
Foster KR, Moulder JE (2013) “
Wi-Fi and Health: Review of Current Status of Research,”
Health Physics, Volume 105, Pages 561-575.
Foster KR, Moulder JE (2018) “
Response to Pall, ‘Wi-Fi is an Important Threat to Human Health’,”
Environmental Research, Volume 445-447, Pages 445-447.
Khurana VG, Moulder JE, Orton CG (2008) “
There
is Currently Enough Evidence and Technology Available to Warrant Taking
Immediate Steps to Reduce Exposure of Consumers to Cell-Phone-Related
Electromagnetic Radiation,”
Medical Physics, Volume 35, Pages 5203-5206.
Moulder JE, Foster KR, Erdreich LS, McNamee JP (2005) “
Mobile Phones, Mobile Phone Base Stations and Cancer: A Review,”
International Journal of Radiation Biology, Volume 81, Pages 189-203.
Pall ML (2018) “
Wi-fi is an Important Threat to Human Health,”
Environmental Research, Volume 164, Pages 405-416.
Sheppard AR, Swicord ML, Balzano Q (2008) “
Quantitative evaluation of mechanisms of radiofrequency interactions with biological molecules and processes,”
Health Physics, Volume 95, Pages 365-396.
Vecchia P, Matthes R, Ziegelberger G, Lin J, Saunders R, Swerdlow A (2009) “
Exposure to High Frequency Electromagnetic Fields, Biological Effects and Health Consequences (100 kHz – 300 GHz),” Munich: International Commission on Non-ionizing Radiation Protection.
Vila, J, Turner MC, Gracia-Lavedan E, Figuerola J, Bowman JD, Kincl L, Richardson L, Benke G, Hours M, Krewski D, McLean D, Parent M-E, Sadetzki S, Schlaefer K, Schlehofer B, Schuz J, Siemiatycki J, Tongeren M, Cardis, E (2018)
Occupational exposure to high-frequency electromagnetic fields and brain tumor risk in the INTEROCC study: An individualized assessment approach.
Environment International, Volume 119, Pages 353-365.